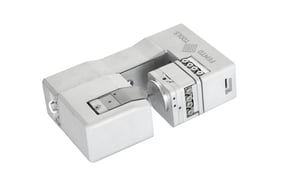
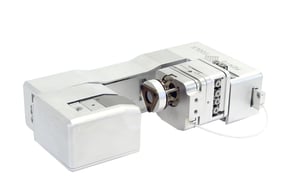
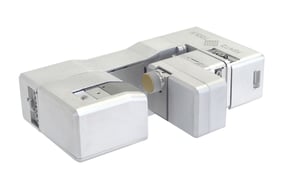
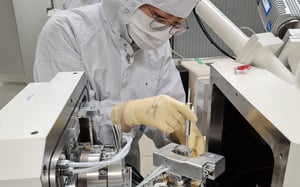
The NMT04 NanoMechanical Testing System stands at the forefront of in-situ SEM/FIB nanoindentation technology. Leveraging FemtoTools' exclusive MEMS technology, it provides unmatched precision for analyzing materials on the micro- and nanoscale.
With MEMS-based force sensors, the NMT04 boasts exceptional resolution, repeatability, and dynamic response. This system is ideal for nanoindentation of metals, ceramics, thin films, and micro-scale structures like metamaterials and MEMS. It features expandable capabilities via accessories for diverse research fields.
It excels in applications such as micro-compression testing, tensile testing of thin films or nanowires, and fracture testing of micro-beams. This provides precise quantification of plastic deformation, crack growth, and fracture toughness. Its compact design fits most commercial SEMs, enabling comprehensive correlative analysis with techniques like EBSD and EDX.
Lorem ipsum dolor sit amet, consetetur sadipscing elitr, sed diam nonumy eirmod tempor invidunt ut labore et dolore magna aliquyam erat, sed diam voluptua. At vero eos et accusam et justo duo dolores-
At vero eos et accusam et justo duo dolores et ea rebum. Stet clita kasd gubergren, no sea takimata sanctus est Lorem ipsum dolor sit amet. Lorem ipsum dolor sit amet, consetetur sadipscing elitr, sed diam nonumy eirmod tempor invidunt ut labore et dolore magna aliquyam erat.
Stet clita kasd gubergren, no sea takimata sanctus est Lorem ipsum dolor sit amet. Lorem ipsum dolor sit amet, consetetur sadipscing elitr, sed diam nonumy eirmod tempor invidunt ut labore et dolore.
Key Features
- 01 Unmatched Dynamic Range
- 02 True Displacement Control
- 03 Open Measurement Frame

Micro-Electro-Mechanical System (MEMS)-based force sensors have an unmatched dynamic range of up to 100 kHz, thanks to their exceptionally small mobile mass and high stiffness. Moreover, the fine fabrication tolerances of MEMS achieve the lowest possible noise floor, reaching down to 500 pN.
up to 100 kHz |
down to 500 pN |

Displacement-controlled nanoindentation is essential for measuring and comparing mechanical properties at consistent indentation depths, ensuring the probing of equivalent material volumes.
Native displacement control |
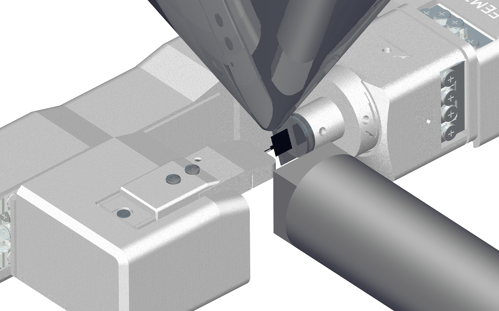
The NMT04 is specially-designed to work seamlessly with Electron Backscatter Diffraction (EBSD) or Scanning Transmission Electron Microscopy (STEM) detectors inside the SEM. Its open measurement frame enables simultaneous observation and analysis of the evolution of the microstructure of the sample during mechanical testing.
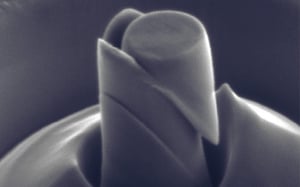
Micro-Pillar Compression
In-situ micro-compression experiments in the SEM allow direct observation of plastic deformation during testing. Displacement control allows deformation to precise strains and preserves deformed structures for subsequent analysis.
READ MORE ->
Read More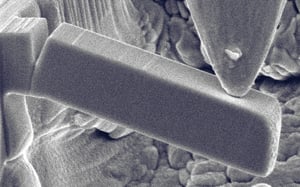
Micro-Cantilever Testing
In-situ bending tests, using micro-cantilevers prepared by lithography or FIB, offer insight into materials’ performance under complex shear stress and fracture conditions – allowing direct crack path observation with stress-strain analysis under controlled displacements.
READ MORE ->
Read More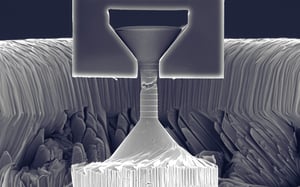
Micro-Tensile Testing
Tensile testing is the gold standard for assessing materials’ ductility and work hardening behavior. Micro-tensile testing extends this technique to the microstructural scale, facilitating the measurement of properties of individual phases, grains, or interfaces.
READ MORE ->
Read More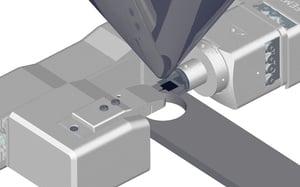
Simultaneous Mechanical Testing with STEM/EBSD
FemtoTools SEM nanoindenters allow for real-time mechanical testing and microscopy (STEM/EBSD/DIC), capturing both crystallographic evolution and force reactions.
READ MORE ->
Read More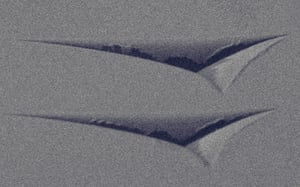
Nanoindentation
Nanoindentation assesses the local mechanical behavior of a material by pressing a sharp tip into its surface and measuring the force needed to create an indent. This technique measures local mechanical properties (including hardness, elastic modulus, strain rate sensitivity, and others) directly from the material's response.
READ MORE ->
Read More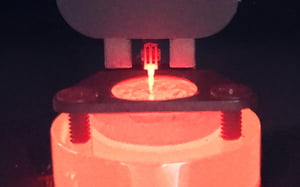
High-Temperature Testing
Temperature changes the mechanical behavior of materials. It is crucial for assessing operando mechanical properties, under conditions close to their target application. Using precision MEMS heating to achieve rapid temperature matching and thermal control, stable measurements can be performed even at high temperatures.
READ MORE ->
Read More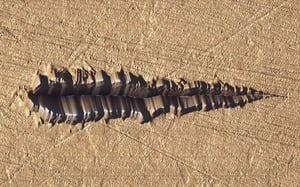
Scratch Testing
Scratch is a critical method for assessing materials’ adhesion, hardness, and resistance to wear - especially for coatings. It provides quantitative data on material durability and performance by scribing a hard tip across the surface and measuring the required force and the resulting topography.
READ MORE ->
Read More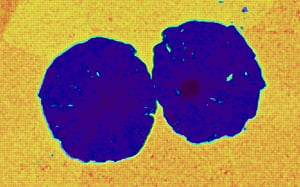
Mechanical Microscopy
Nanoindentation mapping, or mechanical microscopy, employs a nanoindenter in a microscope-like manner. It enables the mapping of mechanical properties of intricate microstructures in minutes, thanks to the integration of rapid indentation speeds and precise spatial resolution.
READ MORE ->
Read More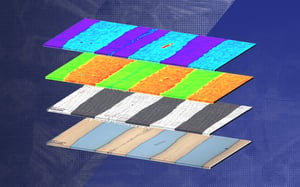
Correlative Mechanical Microscopy
Correlative mechanical microscopy merges nanoindentation with additional microscopy methods. This integration of various data layers enables precise phase identification by including elemental or crystallographic analysis in the mechanical measurement.
READ MORE ->
Read More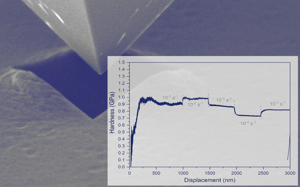
Strain-Rate Control
Time, like temperature, is a key parameter for materials deformation. Speed determines the active deformation mechanism. Strain-rate control during testing achieves the most consistent results and allows exploring the material's response from creep to impact, even within a single indent.
READ MORE ->
Read MoreAccessories
- 01 High-Temperature Module
- 02 Scratch Testing Module
- 03 Rotation Stage Module
- 04 Nano-Tensile Testing Chip
- 05 Microforce Sensing Probes
- 06 Two-Axis Microforce Probes
- 07 High-Temperature Probes
FT-SEM-HT04
High-Temperature Module
The high-temperature module enables mechanical testing, such as nanoindentation, micro-pillar compression, micro-cantilever bending, micro-tensile testing at controlled temperatures up to 800°C. In combination with our microforce sensing probes featuring tip heating, controlled isotherm testing is possible.
FT-ST04
Scratch Testing Module
Combining an exchangeable sample stage with a piezoscanner and a FemtoTools 2-Axis Microforce Sensing Probe enables advanced techniques, such as nano-scratch and nano-wear testing, as well as SPM imaging, by facilitating the in-plane movement of the sample while simultaneously applying a normal force.
FT-SEM-ROT*
Rotation Stage
The rotation stage permits the rotary alignment of samples to the indenter tip, making it possible to test multiple samples in one session by using the rotation stage as a sample revolver. Additionally, it simplifies correlative nanomechanical testing by allowing the sample to be rotated easily between the indentation and imaging positions.
*Currently not available in the USA and Japan.
FT-TT02
Nano-Tensile Testing Chip
This MEMS-based Tensile Testing Chip consists of a movable body that is suspended by four flexures within an outer, fixed frame. It enables the tensile testing of samples thin enough to be electron-transparent, allowing for simultaneous imaging with Scanning Transmission Electron Microscopy (STEM) or Transmission Kikuchi Diffraction (TKD).
FT-S
Microforce Sensing Probes
The FT-S Microforce Sensing Probes are sensors capable of measuring forces from sub-nanonewton to 2 newtons along the sensor’s probe axis. They are available with a wide variety of tip materials and geometries, including diamond Berkovich, cube corner, flat punch, wedge, conical, and more. Specialized versions, featuring 2-axis force sensing or tip heating, are also available.
FT-S
Two-Axis Microforce Sensing Probes
The FT-S Two-Axis Microforce Sensing Probes are Micro-Electro Mechanical Systems (MEMS) based multi-axis microforce sensors capable of simultaneous measuring forces in the two in-plane directions. The primary application for these sensors is nano-scratch, nano-tribology and nano-wear testing.
FT-S
High-Temperature Microforce Sensing Probes
The FT-S High-Temperature Microforce Sensing Probes are Micro-Electro Mechanical Systems (MEMS) based microforce sensors capable of measuring forces from 200mN to 2 µN. Furthermore, with the monolithically integrated tip heater, the temperature of the nanoindenter tip can be accurately matched to the temperature of the sample, enabling isothermal testing conditions.
X. Mao et al., 'Mechanical characterizations of η′-Cu6(Sn, In)5 intermetallic compound solder joint: Getting prepared for future nanobumps', Journal of Materials Research and Technology, vol. 30, pp. 1136-1147, 2024.
B. Wang, J. Ke, and J. Zhang, ‘Thermomechanical properties of metal-organic framework HKUST-1 crystals’, Journal of Materials Chemistry A, 2024.
B. E. Rhodes et al., ‘Deformation mechanisms and defect structures in Heusler intermetallic MnCu2Al’, Acta Materialia, p. 119711, 2024.
T. Loaiza, T. Fischer, R. P. Babu, and P. Hedström, ‘Micromechanical response of dual-hardening martensitic bearing steel before and after rolling contact fatigue’, Journal of Materials Research and Technology, 2024
S. Khademorezaian et al., ‘Extreme rejuvenation of a bulk metallic glass at the nanoscale by swift heavy ion irradiation’, Journal of Alloys and Compounds, vol. 980, p. 173571, 2024.
D. Hernández-Escobar, A. Slagter, S. P. Amarillo, and A. Mortensen, ‘Room-temperature strength of the interfacial bond between silica inclusions and iron’, Acta Materialia, vol. 263, p. 119502, 2024.
M. Freisinger, A. Trausmuth, R. Hahn, and E. Badisch, ‘Influence of the evolution of near-surface rail wheel microstructure on crack initiation by micro-bending investigations’, Proceedings of the Institution of Mechanical Engineers, Part F: Journal of Rail and Rapid Transit, vol. 238, no. 2, pp. 249–255, Feb. 2024, doi: 10.1177/09544097231191550.
M. Freisinger, M. R. Ripoll, and R. Hahn, ‘Unveiling the mechanical properties of near-surface microstructures in tribological contacts via in-situ micro-bending tests’, Tribology International, vol. 191, p. 109190, 2024.
M. Freisinger, S. Fellner, C. Gammer, H. Riedl, and R. Hahn, ‘Stratified surface layers affecting crack propagation in wheel-rail contacts’, Tribology International, vol. 192, p. 109319, 2024.
A. Dhal et al., ‘Multimodal and multiscale strengthening mechanisms in Al-Ni-Zr-Ti-Mn alloy processed by laser powder bed fusion additive manufacturing’, Materials & Design, vol. 237, p. 112602, 2024.
L. Borasi and A. Mortensen, ‘On the slip burst amplitude cutoff in dislocation-rich microcrystals’, Acta Materialia, vol. 264, p. 119582, 2024.
L. Borasi and A. Mortensen, ‘Intermittent tensile deformation of silver microcastings: Influence of the strain rate’, Scripta Materialia, vol. 239, p. 115820, 2024.
P. Agrawal et al., ‘Ion irradiation and examination of Additive friction stir deposited 316 stainless steel’, Materials & Design, p. 112730, 2024.
Y. Zheng, X. Li, P. Liu, Y. Chen, and C. Guo, ‘The armor of the Chinese sturgeon: A study of the microstructure and mechanical properties of the ventral bony plates’, Micromachines, vol. 14, no. 2, p. 256, 2023.
W. Zhang et al., ‘Suppressed Size Effect in Nanopillars with Hierarchical Microstructures Enabled by Nanoscale Additive Manufacturing’, Nano Lett., vol. 23, no. 17, pp. 8162–8170, Sep. 2023, doi: 10.1021/acs.nanolett.3c02309.
J. Zhang et al., ‘Experimental Observation of Highly Anisotropic Elastic Properties of Two-Dimensional Black Arsenic’, Nano Lett., vol. 23, no. 19, pp. 8970–8977, Oct. 2023, doi: 10.1021/acs.nanolett.3c02515.
J. Zhang, J. Ke, B. Wang, and X. Chen, ‘Plastic Avalanches in Metal–Organic Framework Crystals Due to the Dynamic Phase Mixing’, ACS Appl. Mater. Interfaces, vol. 15, no. 47, pp. 54692–54701, Nov. 2023, doi: 10.1021/acsami.3c13480.
J. Zhang, J. Ke, B. Wang, and X. Chen, ‘Plastic avalanches in metal-organic framework crystals’. arXiv, Jul. 27, 2023. Accessed: Mar. 22, 2024.
L. Zauner et al., ‘Influence of Si segregates on the structural evolution, mechanical properties, and high-temperature fracture toughness of Cr-Si-B2\pmz coatings’, Journal of Alloys and Compounds, vol. 958, p. 170354, 2023.
A. Turki, B. Guelorget, V. Optasanu, M. François, F. Herbst, and N. Njah, ‘Microstructure and activation volume of a Cu-6 wt% Zn brass processed by equal channel angular pressing’, Journal of Alloys and Compounds, vol. 968, p. 171974, 2023.
S. Taheriniya et al., ‘Additively manufactured equiatomic CoCrFeMnNi high entropy alloy: Precipitation-induced heterogeneity by mechano-chemical coupling’, Journal of Alloys and Compounds, vol. 938, p. 168514, 2023.
A. I. Slagter, ‘Micromechanics of oxide inclusions in ferrous alloys’, EPFL, 2023. Accessed: Mar. 22, 2024.
A. Slagter, J. Everaerts, L. Deillon, and A. Mortensen, ‘Strong silicon oxide inclusions in iron’, Acta Materialia, vol. 242, p. 118437, 2023.
R. R. P. PUROHIT and B. RHODES, ‘Shifts’, 2023, Accessed: Mar. 22, 2024.
C. Parish, Y. Kato, and S. Melton, ‘FUSION MATERIALS SEMIANNUAL PROGRESS REPORT FOR THE PERIOD ENDING JUNE 30, 2023’, Oak Ridge National Laboratory (ORNL), Oak Ridge, TN (United States), 2023. Accessed: Mar. 22, 2024.
M. J. Parente and B. Sitharaman, ‘Synthesis and Characterization of Carbon Microbeads’, ACS Omega, vol. 8, no. 37, pp. 34034–34043, Sep. 2023, doi: 10.1021/acsomega.3c05042.
A. Michelson, T. J. Flanagan, S.-W. Lee, and O. Gang, ‘High-strength, lightweight nano-architected silica’, Cell Reports Physical Science, vol. 4, no. 7, 2023, Accessed: Mar. 22, 2024.
T. Matsuda, S. Yamada, S. Okubo, and A. Hirose, ‘Antioxidative copper sinter bonding under thermal aging utilizing reduction of cuprous oxide nanoparticles by polyethylene glycol’, J Mater Sci, vol. 58, no. 40, pp. 15617–15633, Oct. 2023, doi: 10.1007/s10853-023-08976-5.
T. Matsuda, R. Seo, and A. Hirose, ‘Highly strong interface in Ag/Si sintered joints obtained through Ag2O–Ag composite paste’, Materials Science and Engineering: A, vol. 865, p. 144647, 2023.
T. Matsuda, K. Hayashi, C. Iwamoto, T. Nozawa, M. Ohata, and A. Hirose, ‘Crack initiation and propagation behavior of dissimilar interface with intermetallic compound layer in Al/steel joint using coupled multiscale mechanical testing’, Materials & Design, vol. 235, p. 112420, 2023.
M. Kagias and J. R. Greer, ‘Morphologically graded scalable nanoarchitected materials’, 2023, Accessed: Mar. 22, 2024.
H. Jiang, Q. Gong, M. Peterlechner, S. V. Divinski, and G. Wilde, ‘Microstructure analysis of a CoCrFeNi high-entropy alloy after compressive deformation’, Materials Science and Engineering: A, vol. 888, p. 145785, 2023.
A. Jelinek, S. Zak, M. J. Cordill, D. Kiener, and M. Alfreider, ‘Nanoscale printed tunable specimen geometry enables high-throughput miniaturized fracture testing’, Materials & Design, vol. 234, p. 112329, 2023.
R. S. Haridas et al., ‘Synergy of tensile strength and high cycle fatigue properties in a novel additively manufactured Al-Ni-Ti-Zr alloy with a heterogeneous microstructure’, Additive Manufacturing, vol. 62, p. 103380, 2023.
R. Hahn et al., ‘Unraveling the superlattice effect for hexagonal transition metal diboride coatings’, Scripta Materialia, vol. 235, p. 115599, 2023.
A. Gumaste et al., ‘A Novel Approach for Enhanced Mechanical Properties in Solid-State Additive Manufacturing by Additive Friction Stir Deposition Using Thermally Stable Al-Ce-Mg Alloy’, JOM, vol. 75, no. 10, pp. 4185–4198, Oct. 2023, doi: 10.1007/s11837-023-06044-6.
C. Fuger et al., ‘Tissue phase affected fracture toughness of nano-columnar TiB 2 + z thin films’, Materials Research Letters, vol. 11, no. 8, pp. 613–622, Aug. 2023, doi: 10.1080/21663831.2023.2204120.
M. Freisinger, L. Zauner, R. Hahn, H. Riedl, and P. H. Mayrhofer, ‘In-situ micro-cantilever bending studies of a white etching layer thermally induced on rail wheels’, Materials Science and Engineering: A, vol. 869, p. 144805, 2023.
A. Dong et al., ‘The effect of sample thickness on micro-mesoscale tensile properties of 304SS, HT-9, and CuCrZr’, Journal of Nuclear Materials, vol. 575, p. 154207, 2023.
A. Dhal et al., ‘High-Throughput Investigation of Multiscale Deformation Mechanism in Additively Manufactured Ni Superalloy’, Metals, vol. 13, no. 2, p. 420, 2023.
A. Dhal et al., ‘High-Throughput Investigation of Multiscale Deformation Mechanism in Additively Manufactured Ni Superalloy. Metals 2023, 13, 420’. 2023. Accessed: Mar. 22, 2024.
D. Cheng et al., ‘Freestanding LiPON: from Fundamental Study to Uniformly Dense Li Metal Deposition Under Zero External Pressure’. arXiv, Apr. 11, 2023. Accessed: Mar. 22, 2024.
D. Cheng et al., ‘A free-standing lithium phosphorus oxynitride thin film electrolyte promotes uniformly dense lithium metal deposition with no external pressure’, Nature Nanotechnology, vol. 18, no. 12, pp. 1448–1455, 2023.
D. Cheng, Deepening the Understanding of Lithium Phosphorus Oxynitride and Associated Interfaces via Advanced Electron Microscopy in All-solid-state Thin Film Batteries. University of California, San Diego, 2023. Accessed: Mar. 22, 2024.
L. Borasi, S. Frasca, E. Charbon, and A. Mortensen, ‘The effect of size, orientation and temperature on the deformation of microcast silver crystals’, Acta Materialia, vol. 249, p. 118817, 2023.
L. Borasi, ‘Bringing casting to microfabrication: processing and deformation of microcastings’, EPFL, 2023. Accessed: Mar. 22, 2024.
A. Bahr et al., ‘Oxidation behaviour and mechanical properties of sputter-deposited TMSi2 coatings (TM= Mo, Ta, Nb)’, Journal of Alloys and Compounds, vol. 931, p. 167532, 2023.
P. Agrawal, S. Gupta, A. Dhal, R. Prabhakaran, L. Shao, and R. S. Mishra, ‘Irradiation response of innovatively engineered metastable TRIP high entropy alloy’, Journal of Nuclear Materials, vol. 574, p. 154217, 2023.
L. Zauner et al., ‘Assessing the fracture and fatigue resistance of nanostructured thin films’, Acta Materialia, vol. 239, p. 118260, 2022.
R. N. Quammen and P. F. Rottmann, ‘Investigation of low temperature oxidation behavior of MoNbTaW thin films’, Journal of Alloys and Compounds, vol. 900, p. 163373, 2022.
R. S. Mishra, ‘Abhijeet Dhal1, 2, Saket Thapliyal1, 2, 3, Supreeth Gaddam1, 2, Priyanka Agrawal1, 2 &’, Scientific Reports, vol. 12, p. 18344, 2022.
T. Matsuda et al., ‘Fracture dominant in friction stir spot welded joint between 6061 aluminum alloy and galvannealed steel based on microscale tensile testing’, Materials & Design, vol. 213, p. 110344, 2022.
A. Godet, J. Chretien, K. P. Huy, and J.-C. Beugnot, ‘Micronewton nanofiber force sensor using Brillouin scattering’, Optics Express, vol. 30, no. 2, pp. 815–824, 2022.
Z. Gao, J. Buchinger, N. Koutná, T. Wojcik, R. Hahn, and P. H. Mayrhofer, ‘Ab initio supported development of TiN/MoN superlattice thin films with improved hardness and toughness’, Acta Materialia, vol. 231, p. 117871, 2022.
Z. Gao, ‘Architectural Design of Transition Metal Nitride Thin Films for Improved Mechanical or Electrical Properties’, PhD Thesis, Technische Universität Wien, 2022. Accessed: Mar. 22, 2024.
J. Everaerts, A. Slagter, and A. Mortensen, ‘A method for cleaning flat punch diamond microprobe tips’, Micron, vol. 155, p. 103217, 2022.
J. Domitner et al., ‘Microstructure Characterization of Nickel Matrix Composite Reinforced with Tungsten Carbide Particles and Produced by Laser Cladding’, Adv Eng Mater, vol. 24, no. 11, p. 2200463, Nov. 2022, doi: 10.1002/adem.202200463.
A. Dhal, S. Thapliyal, S. Gaddam, P. Agrawal, and R. S. Mishra, ‘Multiscale hierarchical and heterogeneous mechanical response of additively manufactured novel Al alloy investigated by high-resolution nanoindentation mapping’, Scientific Reports, vol. 12, no. 1, p. 18344, 2022.
A. Bahr et al., ‘Non-reactive HiPIMS deposition of NbCx thin films: Effect of the target power density on structure-mechanical properties’, Surface and Coatings Technology, vol. 444, p. 128674, 2022.
S. Taheriniya et al., ‘High entropy alloy nanocomposites produced by high pressure torsion’, Acta Materialia, vol. 208, p. 116714, 2021.
T. Matsuda et al., ‘Fracture behavior of thermally aged Ag–Cu composite sinter joint through microscale tensile test coupled with nano X-ray computed tomography’, Materials & Design, vol. 206, p. 109818, 2021.
T. Glechner et al., ‘Structure and mechanical properties of reactive and non-reactive sputter deposited WC based coatings’, Journal of Alloys and Compounds, vol. 885, p. 161129, 2021.
T. Matsuda, T. Sano, M. Munekane, M. Ohata, and A. Hirose, ‘Microscale tensile test of galvannealed steel/aluminum dissimilar joint for estimation of intrinsic interfacial strength’, Scripta Materialia, vol. 186, pp. 196–201, 2020.
D. R. Cayll, I. S. Ladner, J. H. Cho, S. K. Saha, and M. A. Cullinan, ‘A MEMS dynamic mechanical analyzer for in situ viscoelastic characterization of 3D printed nanostructures’, Journal of Micromechanics and Microengineering, vol. 30, no. 7, p. 075008, 2020.
S. Taheriniya et al., ‘Mechano-Chemical Coupling, Heterogeneous Segregation and Nano-Precipitation in Additively Manufactured Equatomic COCrFeMnNi High Entropy Alloys’, Heterogeneous Segregation and Nano-Precipitation in Additively Manufactured Equatomic COCrFeMnNi High Entropy Alloys.
T. Matsuda, R. Seo, and A. Hirose, ‘Highly Strong Ag/Sio2/Si Sintered Interfaces Assisted by Incorporation of in Situ Generated Ag Nanoparticles’, Available at SSRN 4104269, Accessed: Mar. 22, 2024.
J. Ke, Z. Yao, and J. Zhang, ‘Interphase Mechanical Behaviours of Graphene-Copper Nanocomposites: a Study by In-Situ Nanoindentations and Molecular Dynamics Simulations’, Available at SSRN 4754112, Accessed: Mar. 22, 2024.
S. Kagerer et al., ‘The micromechanical behavior of magnetron sputtered TiN/Nb Multilayers’, Nb Multilayers, Accessed: Mar. 22, 2024.